Why the Earth Has Fewer Species Than We Think
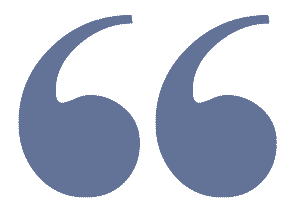
This is not supposed to happen. The revolution is the realization that it does. It happened to the nautilus. And it happens to you and me.
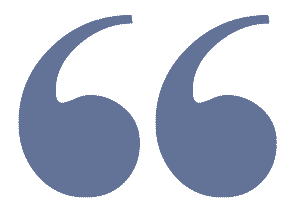
A primer on the epigenome.
In 2012, I ran a trip sampling the nautilus populations along Australia's Great Barrier Reef explicitly to see if nautiluses living on marine protected areas of the reef are as rare as from places where they are fished for their pretty shells (such as in the Philippines and Indonesia). Work along the Great Barrier Reef in the 1990s had shown that two different and accepted species are present. One, Nautilus pompilius, is the most widespread of all the nautiluses across their vast Pacific and Indian Ocean range. The second, Nautilus stenomphalus, is found only on the Great Barrier Reef. It differs from the more common N. pompilius in having a hole right at the center of its shell. (In N. pompilius, there is a thick calcareous plug.) There are also marked differences in shell coloration and pattern of stripes on the shell. But when the Australian species was first brought up from its 1,000-foot habitat alive, in the late 20th century, scientists were astonished to find that N. stenomphalus has markedly different anatomy as well on its thick "hood", a large fleshy area that protects the interior guts and other anatomical soft parts when the animal pulls into its shell. In N. pompilius the hood is covered with low bumps of flesh, like warts. Meanwhile the N. stenomphalus hood is covered with a forest of brushy projections that rise above the hood like a thick carpet of twiggy moss, or tiny trees of flesh; the coloration of the hood is also radically different.
The 2012 trip was to sample the DNA of the two "species" as well as to better understand how many nautiluses live on a given area of seafloor. We caught 30 nautiluses over nine days, snipped off a one-millimeter-long tip of one of each nautilus' 90 tentacles, and returned all back to their habitats alive (if cranky). All the samples were later analyzed in the large machines that read DNA sequences, and to our complete surprise we found that the DNA of N. pompilius and the morphologically different N. stenomphalus was identical. No genetic difference, yet radically different morphology. The best way to interpret this is to go back to one of the most useful analogies in evolution: of a ball rolling down a slope composed of many gullies. Which gully the ball rolls down (corresponding to the ultimate anatomy or "phenotype" of the grown animal) is controlled by the direction of the push of the ball. In evolution, the ultimate morphological fate of an organism is caused by some aspect of the environment the organism is exposed to early in life - or, in the case of the nautiluses, while they slowly develop in their large egg over the course of an entire year before hatching. Perhaps it is a difference in temperature. Perhaps it is forces that the embryo encounters prehatching, or when newly hatched, the small nautiluses (one inch in diameter, with eight complete chambers) find different food, or perhaps they are attacked and survive, i.e., have two different kinds of predators. That's why N. pompilius and N. stenomphalus are not two species. They are a single species with epigenetic forces leading to the radically different shell and soft parts. Increasingly it appears that perhaps there are fewer, not more, species on Earth than science has defined.
More and more, biologists are discovering that organisms thought to be different species are, in fact, but one. A recent example is that the formerly accepted two species of giant North American mammoths (the Columbian mammoth and the woolly mammoth) were genetically the same but the two had phenotypes determined by environment.
There are many conflicting uses of the term epigenetics, and this as much as anything has led to great dissension among and between scientists, as well as between scientists and science journalists. This is not an isolated incident: There are many cases in science where specific terms are used in quite different contexts, by different scientists, where the same word takes on disparate meanings; as a consequence, confusion can arise. In the past decade alone, there have been an increasing number of books, popular articles, and scientific reviews concerning epigenetics and in them there has been a diversity of meanings and ways that the word has been used. (And, according to many critics, overused.)
The origin of the word comes from British biologist Conrad Waddington, for whom epigenetics was the study of how "genotype" (the sum of genes contained by an organism) is translated into "phenotype, the actual physical manifestation of the organism, as well as its various and specific chemical properties and productions and, as we increasingly know, its behaviors. But to other scientists, there is a far more specific sense to the term: Epigenetics is the study of heritable gene functions that are passed on from one reproducing cell to another, be that to a somatic (body) cell or to a germ cell (sperm or ovum), which does not involve a change to the original DNA sequence. It is the latter case that can lead to major evolutionary change. During the process of "meiosis", the replication of cells (sperm and eggs) in sexually reproducing organisms, information is put into the sperm or ovum that will, like some exotic secret writing, become readable only after fertilization.
Epigenetics looks to understand how a specific cell at a specific time in a specific anatomical place “knows†how to change into something quite different.
Epigenetics (or heritable epigenetics, or neo-Lamarckism) is a series of different processes that can cause evolutionary changes as well as dictate how organisms develop from a single fertilized egg (in the case of sexually reproducing organisms, at least) to what we look like as adults. Some say it’s just a minor tweak of already understood processes and that it's of little importance in the broader scheme of evolutionary change or the past or even future history of life. But to others epigenetics, while still poorly understood, is potentially of far greater importance than mainstream evolutionary theory, and mainstream evolutionists have heretofore accepted that. To a few, its ongoing discovery is causing an unfolding scientific revolution. But the discoveries have not happened evenly among the many fields within what we call "biology". The great breakthroughs have mainly been studies looking at cells, and the molecules within cells, including DNA and RNA and other aspects of genetics. But to date there has been little if any progress in tying epigenetic change to the many events evidenced by fossils and the fossil record.
In genetics, genes are disrupted by mutations and permanently changed. Epigenetic effects take place when single genes along a long strand of DNA become "polluted" with very small molecules, which each attach to only a single small site along the long DNA molecule. This can cause a gene that was actively in use - such as one dictating the production of a specific protein - to become blocked from its normal activity. That protein is no longer made. But sometimes one single such block can affect the normal operating of hundreds of genes, such as when a master control gene (called a Hox gene) is inadvertently turned off. Because Hox genes control hundreds of other genes by telling them when and where to turn on and off, a single epigenetic change to that gene now affects a vast number of other genes. Hox genes dictate the building of organs, limbs, skin, and every part of a developing organism. Causing a Hox gene to turn off can have profound biological effects far greater than any single mutation. In this way, epigenetic change can radically and quickly transform the anatomy of an organism - for better or worse.
In epigenetics, genes that are inactive (silent) thus can be awakened and begin causing biological effects in an organism by environmental stimuli that would not happen if those environmental stimuli were absent. They are not necessarily permanent changes: The small attaching molecules are not permanently welded in place; DNA has long ago evolved the means to repair itself, including the removal of these bad molecules. Thus, in most cases epigenetic changes that affect us have no effect on our offspring. But sometimes these epigenetic changes do get passed on through eggs and sperm.
The study of epigenetics really comes down to observing two types of epigenetic changes. The first type of changes are the "normal" epigenetic changes that organisms go through, honed by natural selection. For instance, every cell in our bodies contains all the necessary information to become one of the many specific kinds of cells necessary to keep us alive, such as the nerve cells, muscle cells, and the many other highly specialized cell types that are necessary for living. Every cell contains the DNA information to become any or all. But it does. But they do not. The science involved in epigenetics looks to understand how it is that a specific cell at a specific time in a specific anatomical place "knows" how to change into something quite different according to time, place, and function. But the changes are "foreseen" by the organism and beneficial.
The second kind of epigenetic change causes unforeseen modification to an organism without altering the genetic coding for specific genes, but it also passes on these changes. It can cause change ranging from minor to profound, and can be heritable. "Lamarckian" change is where something encountered in its environment, and not necessarily expected in the life of an organism, causes chemical changes to the DNA through the addition of tiny molecules, or through a shape change of the scaffolding that holds the twisted DNA molecules in specific shapes. Other kinds of epigenetic change can also be caused by the actions of small RNA molecules responding to some kind of external environmental change.
Each of these can change how genes act by turning genes on or off. This can include some of the most important genes for our lives, the ones that affect our behavior through the rate at which hormones dictating emotions are regulated and supplied.
Here is a fuller description of the most important identified means by which epigenetic changes are produced:
The second kind of epigenetic change causes unforeseen modification to an organism without altering the genetic coding for specific genes, but it also passes on these changes. It can cause change ranging from minor to profound, and can be heritable. "Lamarckian" change is where something encountered in its environment, and not necessarily expected in the life of an organism, causes chemical changes to the DNA through the addition of tiny molecules, or through a shape change of the scaffolding that holds the twisted DNA molecules in specific shapes. Other kinds of epigenetic change can also be caused by the actions of small RNA molecules responding to some kind of external environmental change.
Each of these can change how genes act by turning genes on or off. This can include some of the most important genes for our lives, the ones that affect our behavior through the rate at which hormones dictating emotions are regulated and supplied.
Here is a fuller description of the most important identified means by which epigenetic changes are produced:
Methylation is the addition of very short chains of carbon, oxygen, and hydrogen to particular nucleotides in DNA, which typically silences gene activity.
Histone modification involves the chemicals (histones) that serve like support structures for a DNA molecule. They can cause the shape of the DNA to change by making it more or less packed on itself. When they are modified by the addition of one of several small chemical molecules (again, a methyl molecule, which is the tiny molecule with a single carbon atom accompanied by hydrogen atoms), as well as additional small chemical groups composed of only a few atoms, they are added on to the much larger histones, thus changing the overall shape of this chemical "scaffolding" that holds the DNA molecule within the cell. When so packed, the DNA is harder to get to by the small molecules of RNA trying to read the code, and they go to the cell's protein factories, such as ribosomes, where proteins called for by the DNA are actually built.
We can pass on the physical and biological effects of our good or bad habits and even the mental states acquired during our lives.
A third kind of change is caused by tiny RNA molecules (RNAi) affecting the chromatin (the histones) described above. In fact, a diverse assemblage of different-length RNA molecules are now known to be regulators of gene expression, as well as being used in genome defense against foreign genetic elements such as attacks on a cell by a virus. Small RNAs modify the shape of the chromatin structure and can stop (silence) the process known as transcription, where a gene dictates which protein should be built.
Sometimes an epigenetic change causes a protein not to be made. Sometimes it causes the making of a new protein that would not otherwise occur. Sometimes, and most important, it causes a regulator gene (essentially the “general contractor†coordinating all of the cells on the body's busy construction projects) to walk off the job entirely. This causes huge changes far beyond what any single mutation could do. Such changes affecting an individual can then be passed to the next generation. The methyl molecules are not physically passed on to the next generation, but the propensity for them to attach in the same places in an entirely new life-form (a next-generation life-form) is. This methylation is caused by sudden traumas to the body, such as poisoning, fear, famine, and near-death experience. None of these events come from small methyl molecules, but they cause small methyl molecules already in the body to swarm onto the entire DNA in the body at specific and crucial sites. These acts can have an effect not only on a person's DNA but on the DNA of their offspring. The dawning view is that we can pass on the physical and biological effects of our good or bad habits and even the mental states acquired during our lives.
This is a stark change from the theory of evolution through natural selection. Heritable epigenetics is not a slow, thousand-year process. These changes can happen in minutes. A random hit to the head by an enraged lover. A sick, sexually abusive parent. Breathing in toxic fumes. Coming to God in religious ecstasy. All can change us, and possibly change our children as a consequence.
In heritable epigenetics, we pass on the same genome, but one marked (mark is the formal term for the place that a methyl molecule attaches to one nucleotide, a rung in the ladder of DNA) in such a way that the new organism soon has its own DNA swarmed by these new (and usually unwelcome) additions riding on the chromosomes. The genotype is not changed, but the genes carrying the new, sucker-like methyl molecules change the workings of the organism to something new, such as the production (or lack thereof) of chemicals necessary for our good health, or for how some part of the body is produced. Thus, the young of an epigenetically modified parent can be radically different in phenotype from the parent. Phenotype is the physical manifestation of genotype, such as hair and eye color or body dimensions in a human - or of IQ and brain functioning. Sometimes these changes allow the young organism to deal with environments that were intolerable to the parents. Sometimes these changes rapidly create new species. But sometimes the consequences can be fatal and the changes can be passed on to yet a subsequent generation. In other words, a young child could suffer from the sins of a grandfather.
There remains a great deal of dispute as to the relative importance of epigenetics, the extent to which it is heritable, and even if there is anything actually novel compared to the classical, establishment view that random mutation is the prime fuel of evolutionary change and to the resulting record that is the history of life. Much of this discourse comes from the ongoing belief that what is called "reprogramming" makes the epigenetic additions of methyl molecules attached to DNA a nonfactor - in that they are erased at fertilization. It has long been "truth" that the epigenome (the complement of chemicals that modify the expression and function of the organism's genes, such as the methyl molecules that can glom onto specific genes during the life of the organism due to some environmental change) of the parent is reprogrammed (all epigenetic traces removed) twice: once during the formation of the gamete itself (the unfertilized egg, or a sperm waiting around to fertilize an egg) and secondly at conception. Erase and erase again. But now experiments definitively show that some of the chemicals added during the life of an organism do leave information in such a way that the offspring has their genes quickly modified in the same way that the parents did. The same places on the long DNA molecules of the newly born (or even the "not-yet" born) get the same epigenetic add-ons that one or both of the parents had. This is not supposed to happen. The revolution is the realization that it does. It happened to the nautilus. And it happens to you and me.
Peter Ward is a professor of Biology and Earth and Space Sciences at the University of Washington. He is the author of In Search of Nautilus and The Flooded Earth: Our Future in a World Without Ice Caps. He is beloved by his family, students, and dog.
Sometimes an epigenetic change causes a protein not to be made. Sometimes it causes the making of a new protein that would not otherwise occur. Sometimes, and most important, it causes a regulator gene (essentially the “general contractor†coordinating all of the cells on the body's busy construction projects) to walk off the job entirely. This causes huge changes far beyond what any single mutation could do. Such changes affecting an individual can then be passed to the next generation. The methyl molecules are not physically passed on to the next generation, but the propensity for them to attach in the same places in an entirely new life-form (a next-generation life-form) is. This methylation is caused by sudden traumas to the body, such as poisoning, fear, famine, and near-death experience. None of these events come from small methyl molecules, but they cause small methyl molecules already in the body to swarm onto the entire DNA in the body at specific and crucial sites. These acts can have an effect not only on a person's DNA but on the DNA of their offspring. The dawning view is that we can pass on the physical and biological effects of our good or bad habits and even the mental states acquired during our lives.
This is a stark change from the theory of evolution through natural selection. Heritable epigenetics is not a slow, thousand-year process. These changes can happen in minutes. A random hit to the head by an enraged lover. A sick, sexually abusive parent. Breathing in toxic fumes. Coming to God in religious ecstasy. All can change us, and possibly change our children as a consequence.
In heritable epigenetics, we pass on the same genome, but one marked (mark is the formal term for the place that a methyl molecule attaches to one nucleotide, a rung in the ladder of DNA) in such a way that the new organism soon has its own DNA swarmed by these new (and usually unwelcome) additions riding on the chromosomes. The genotype is not changed, but the genes carrying the new, sucker-like methyl molecules change the workings of the organism to something new, such as the production (or lack thereof) of chemicals necessary for our good health, or for how some part of the body is produced. Thus, the young of an epigenetically modified parent can be radically different in phenotype from the parent. Phenotype is the physical manifestation of genotype, such as hair and eye color or body dimensions in a human - or of IQ and brain functioning. Sometimes these changes allow the young organism to deal with environments that were intolerable to the parents. Sometimes these changes rapidly create new species. But sometimes the consequences can be fatal and the changes can be passed on to yet a subsequent generation. In other words, a young child could suffer from the sins of a grandfather.
There remains a great deal of dispute as to the relative importance of epigenetics, the extent to which it is heritable, and even if there is anything actually novel compared to the classical, establishment view that random mutation is the prime fuel of evolutionary change and to the resulting record that is the history of life. Much of this discourse comes from the ongoing belief that what is called "reprogramming" makes the epigenetic additions of methyl molecules attached to DNA a nonfactor - in that they are erased at fertilization. It has long been "truth" that the epigenome (the complement of chemicals that modify the expression and function of the organism's genes, such as the methyl molecules that can glom onto specific genes during the life of the organism due to some environmental change) of the parent is reprogrammed (all epigenetic traces removed) twice: once during the formation of the gamete itself (the unfertilized egg, or a sperm waiting around to fertilize an egg) and secondly at conception. Erase and erase again. But now experiments definitively show that some of the chemicals added during the life of an organism do leave information in such a way that the offspring has their genes quickly modified in the same way that the parents did. The same places on the long DNA molecules of the newly born (or even the "not-yet" born) get the same epigenetic add-ons that one or both of the parents had. This is not supposed to happen. The revolution is the realization that it does. It happened to the nautilus. And it happens to you and me.
Peter Ward is a professor of Biology and Earth and Space Sciences at the University of Washington. He is the author of In Search of Nautilus and The Flooded Earth: Our Future in a World Without Ice Caps. He is beloved by his family, students, and dog.
[Removed]